Redox Reactions
SECTION 1 : REDOX REACTIONS
Oxidation and reduction are complementary to each other one can not take place alone. So both oxidation and reduction will take place simultaneously. It is obvious that if a substance takes electrons there must be another substance to give up these electrons. The reaction which involve oxidation and reduction are called redox reactions. A redox reaction can be split into two half reactions namely oxidation half reaction (where oxidation takes place) and reduction half reaction (where reduction takes place).
For Example:
Redox reaction : 2Fe3+ + Sn2+ → 2Fe2+ + Sn4+
Oxidation half reaction : Sn2+ → Sn4+ + 2e–
Reduction half reaction : Fe3+ + e– → Fe2+
SECTION 2 : OXIDATION NUMBER OR OXIDATION STATE
Oxidation number for an element is the arbitrary charge present on one atom when all other atoms bonded to it are removed. The removal of other atoms (dissociation of bonds between them) is done by assuming that the bonds are either ionic or pure covalent.
So, when a bond between two identical atom is broken the bonded electrons are distributed equally between these two atoms. Similarly when a bond between two different atoms is broken (one is more electronegative than other) bonded electrons are assumed to be retained by more electronegative atom.
For example, we consider a molecule of HCl, the Cl atom is more electronegative than H-atom therefore the bonded electrons will go with more electronegative chlorine atom resulting in formation of H+ and Cl– ions. So, oxidation number of H and Cl in HCl are (+1) and (–1) respectively.
The following points are important to determine the oxidation number of an element.
- The oxidation number of an atom in pure elemental form is considered to be zero.
- Sum of all total oxidation states of elements in a neutral compound must be zero.
- Sum of all total oxidation states of elements in a charged ion will be equal to the charge on the ion.
- Oxidation number of any element in simple monoatomic ion will be equal to the charge on that ion for example oxidation number of Na in Na+ is (+1).
- Oxidation number of fluorine in its compound with other elements is always (–1).
- Oxidation number of oxygen is generally –2 but in case of peroxide
and superoxide
oxygen has oxidation number (–1) and
respectively. In a compound OF2 the oxidation number of oxygen is (+2).
- The oxidation number of alkali metals (Na, K) and alkaline earth metals (Ca, Mg) are (+1) and (+2) respectively.
- The oxidation number of halogens is generally (–1) when they are bonded to less electronegative elements.
- Oxidation number of hydrogen is generally (+1) in most of its compound but in case of metal hydride (NaH, CaH2) the oxidation number is hydrogen is (–1).
- The algebraic sum of the oxidation numbers of all the atoms in a neutral compound is zero. In an ion the algebraic sum of oxidation number is equal to the charge on that ion.
SECTION 3 : OXIDISING AND REDUCING AGENT
A substance which undergoes oxidation acts as a reducing agent while a substance which undergoes reduction acts as an oxidising agent. For example we take a redox reaction
Zn + Cu2+ → Zn2+ + Cu
In this reaction Zn is oxidised to Zn2+ so, Zn is the reducing agent and Cu2+ is reduced to Cu so, Cu2+ is the oxidising agent.
Important Points for Identification of Oxidising and Reducing Agent- An elements in its highest possible oxidation state in a compound can behave as an oxidising agent. For example, KMnO4 , K2Cr2O7, H2SO4, HNO3, HClO4.
- An element in its lowest possible oxidation state in a compound it can behave as a reducing agent. For example H2S, FeSO4, SnCl2.
- If element is in its intermediate oxidation state in a compound, it can behave both as reducing as well as oxidising agent. For example, H2O2, HNO2, H2SO3, SO2.
- If a highly electronegative element is in its higher oxidation state in a compound then that compound can behave as a powerful oxidising agent. For example, KClO4, KClO3, KIO3.
- If an electronegative element is in its lowest possible oxidation state in a compound or free state. It can behave as a powerful reducing agent. For example I–, Br–N3– etc.
SECTION 4 : BALANCING OF REDOX REACTIONS
OXIDATION NUMBER METHOD
In this method number of electrons lost in oxidation must be equal to number of electrons gained in reduction. Rules :- Write the skeletal equation of all the reactants and products of the reaction.
- Indicate the oxidation number of each element and identify the elements undergoing change in oxidation number.
- Equalize increase or decrease in oxidation number by multiplying both reactants and products undergone change in oxidation number by suitable integer.
- Balance all atoms other than H and O then balance O atom by adding water molecules to the side short of O-atoms.
- For acidic medium – Balance H atom by adding H+ ion to the side short of H atoms.
- For basic medium – Balance charge by adding OH– ions to make ionic charges equal. Add appropriate number of water molecule to balance H & O atom.
ION-ELECTRON METHOD OR HALF REACTION METHOD
- Write the unbalanced equation in ionic form.
- Separate the equation in to half reactions, i.e., oxidation half reaction and reduction half reaction.
- Balance the atoms other than O and H in each half reaction individually.
- In acidic medium, add H2O to balance O atom and H+ to balance charge.
- Add electrons to whichever side is necessary to balance the oxidation state.
- In basic medium add OH– to balance charge and H2O to side falling short of H atoms.
- Add the two half reactions to achieve the overall reaction and cancel the electrons on each side. In balanced equation the number of atoms and electrical charges must be equal on both sides.
STANDARD REDUCTION POTENTIALS
When the concentrations of the Cu2+ and Zn2+ ions are both 1.0 M, we find that the voltage or EMF of the Daniell cell is 1.10 V at 25°C. This voltage must be related directly to the redox reaction, but how? just as the overall cell reaction can be thought of as the sum of two half-cell reactions, the measured emf of the cell can be treated as the sum of the electrical potentials at the Zn and Cu electrodes. Knowing one of these electrode potentials, we could obtain the other by subtraction. It is impossible to measure the potential of just a single electrode, but if we arbitrarily set the potential value of a particular electrode at zero, we can use it to determine the relative potentials of other electrodes. The hydrogen electrode, serves as the reference for this purpose. Hydrogen gas is bubbled into a hydrochloric acid solution at 25°C. The platinum electrode has two functions. First, it provides a surface on which the dissociation of hydrogen molecules can take place
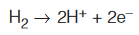
Second, it serves as an electrical conductor to the external circuit. Under standard state conditions (when the pressure of H2 is 1 atm and the concentration of the HCl solution is 1 M), the potential for the reduction of H+ at 25°C is taken to be exactly zero :

The superscript “°” denotes standard-state conditions and E° is standard reduction potential, or the voltage associated with a reduction reaction at an electrode when all solutes are 1 M and all gases are at 1 atm. Thus, the standard reduction potential of the hydrogen electrode is defined as zero. The hydrogen electrode is called the standard hydrogen electrode (SHE).
We can use the SHE to measure the potentials of other kinds of electrodes. For example, consider an electrochemical cell with a zinc electrode and a SHE. In this case the zinc electrode is anode and the SHE is the cathode. We deduce this fact from the decrease in mass of the zinc electrode during the operation of the cell, which is consistent with the loss of zinc to the solution caused by the oxidation reaction :
Zn (s) → Zn2+ (aq) + 2e–
The cell diagram is
Zn (s) | Zn2+ (1 M) || H+ (1 M) | H2 (1 atm) | Pt (s)
As mentioned earlier, the Pt electrode provides the surface on which the reduction takes place. When all the reactants are in their standard states (that is, H2 at 1 atm, H+ and Zn2+ ions at 1 M each, the emf of the cell is 0.76 V at 25°C. We can write the half-cell reactions as follows :

By convention, the standard emf of the cell, E°cell, which is composed of a contribution from the anode and a contribution from the cathode, is given by
E°cell = E°Cathode – E°Anode
where E°Cathode and E°Anode are the standard reduction potential of the cathode and anode respectively. For the Zn-SHE cell, we writeE°cell = E°H+|H2 – E°Zn2+|Zn
0.76 V = 0 – E°Zn2+|Zn
where the subscript H+ | H2 means 2H+ + 2e– → H2 and the subscript Zn2+ | Zn means Zn2+ + 2e– →Zn. Thus the standard reduction potential of zinc, E°Zn2+|Zn, is –0.76 V.